
Research
Length scale matters. Since quantum systems are typically small, we wish to observe and manipulate them on their own terms, with nanometric precision and ultrashort duration, by harnessing laser and optical approaches and riding on the mature technology of electron microscopy.

Although an electron's coupling to radiation is typically weak, when confining photons to micro-resonators they can do much more. Photons with a small mode volume can exert a stronger force compared to a photon in free space, and in addition, traveling in a high-index material allows the photon to coherently amplify the interaction over an extended length, matching its phase velocity to an electron traveling at roughly half the speed of light. Thus, with properly designed optical micro resonators, electrons can be meaningfully entangled to photons, and carry such quantum information with them to a nanometer-size focal point. Our lab aspires to create, measure and utilize such entangled pairs, and to investigate what quantum information they can transfer onward, say to a nanoscopic quantum system. See the paper by Ofer Kfir Physical Review Letters 2019, which opened this field of research, and the first demonstration of electron interaction with an optical microresonator, Nature 2020.
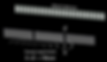
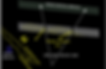
The particle-wave duality sets a length scale to quantum phenomena - the wavelength. We would expect a wave-like behavior for scales near the wavelength and a particle-like behavior when the wavelength is much smaller then anything else. Working with electrons and photons, we can target both scales simultaneously since the wavelengths of a relativistic electron is about a millionth of the that of visible light. Experimenting with cathodoluminescence (CL), which is light emission by an electron beam, we address the role of the quantum measurement and the so-called "quantum collapse" it induces on the wavefunction, alongside questions of coherence and entanglement of electrons, photons, and samples.

Quantum materials such as topological insulators, Weyl semi-metals and two-dimensional materials exhibit unique conduction properties due to the strong interaction of the electron spin with its orbit. These novel transport properties offer quantum materials as the hardware infrastructure for the next generation of logic and sensors.
Our group combines short infrared laser pulses with millimeter-waves (THz frequencies), to drive and to detect and to control the transport within meta-stable states quantum materials. Conducting the experiment in an electron microscope, we can direct ultrashort pulsed electron beams to a nanometeric spot or create a high resolution image snapshot, which can form a movie of the fast nanoscopic dynamics.
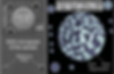
The scattering of soft X-rays and extreme-UV light from materials provides information on the structure of the sample. While X-ray diffraction is standard for determining the atomic positions, a complete reconstruction of the scattering amplitude and phase creates an image, which can be tuned resonantly to enhance a particular contrast, such as elemental, chemical, or magnetic. We use highly coherent sources, such as high-harmonic generation from femto-second lasers, to target a particularly challenging task - the resonant imaging of weakly scattering objects - with an unprecedented resolutions. We have imaged nano-scale magnetic features with sub-wavelength resolution, and observed their dynamics with femto-second precision.
Within this project, our group develops imaging methods and investigates approaches for suppressing the (quantum) shot-noise of the energetic photons. See publications on the first imaging of magnetic features with high harmonics Science Advances 2017, and an unprecedented spatial and temporal resolution for magnetic imaging (even compared with large X-ray sources as Free-electron lasers (FELs) and Synchrotrons, in Nature Communications 2021.
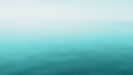